How a slime mold near death packs bacteria to feed the next generation
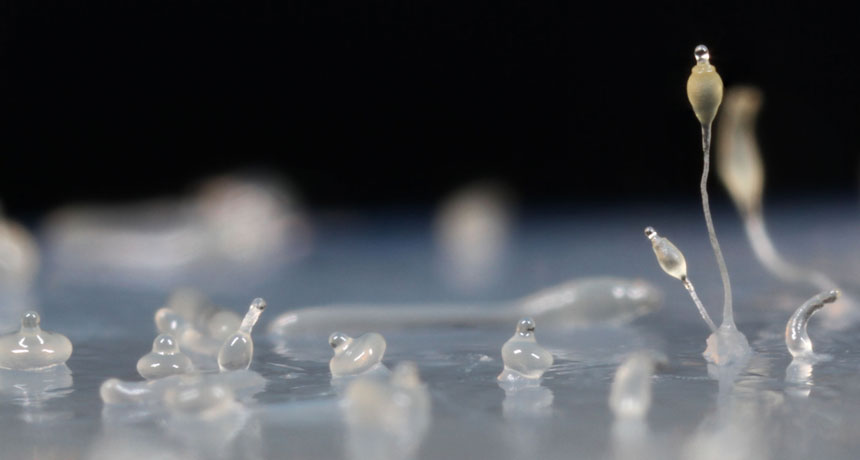
In the final frenzy of reproduction and death, social amoebas secrete proteins that help preserve a starter kit of food for its offspring.
Dictyostelium discoideum, a type of slime mold in soil, eats bacteria. Some wild forms of this species essentially farm the microbes, passing them along in spore cases that give the next generation of amoebas the beginnings of a fine local patch of prey. Tests find that the trick to keeping the parental immune system from killing this starter crop of bacteria is a surge of proteins called lectins, researchers say in the July 27 Science.
Lectins create a different way for the amoebas to treat bacteria: as actual symbionts inside cells, instead of as prey or infections, says study coauthor Adam Kuspa, a molecular cell biologist at Baylor College of Medicine in Houston. In a lab test of this ability, coating other bacteria with lectin derived from a plant allowed bacteria to slip inside cells from mice and survive as symbiotic residents.
The findings mark another chapter in a story that has been upending decades of what people thought they knew about social amoebas eating bacteria. The basic, almost alien, scenario is still true: D. discoideum amoebas, nicknamed Dicty, start life as single cells. When food dwindles, cells come together into a much bigger, multicellular slug-shaped creature with eight to 10 types of cells and the power to crawl. It then develops into something more like a fungus with a stalk holding up a case of spores, which start the next generation of amoebas.
Those casings, scientists once believed, held only spores. “For 70 years, we all thought that Dictyostelium development was sterile,” meaning no bacteria survived among spores, Kuspa says. “If you were not a very good microbiologist and contaminated your amoeba sample, one way to cure them of bacteria was to put them through a cycle of development.”
Then in 2011, researchers discovered that some Dicty strains are “farmers,” routinely packing live bacteria into spore cases, and jump-starting new bacterial livestock with each generation (SN: 2/12/11, p. 11). “That was a shock,” Kuspa says.
Researchers also discovered that the Dicty animal-like slug phase forms an immune system that kills bacteria, even as evidence grew that some bacteria had uses beyond food, such as providing defense chemistry. But how the slug avoided killing its own helpful bacteria was a mystery.
Comparing secretions of Dicty strains carrying bacteria versus strains that don’t showed a “dead-obvious” difference, Kuspa says: more lectins called discoidin A and discoidin C in the carrier forms. A series of tests supplying and withholding the proteins showed big effects on the fates of bacteria. The researchers found that the lectins raise the chances that bacteria can slip inside an amoeba cell and live hidden from immune-system sentinels that purge free-living intruders. That gives the bacteria a chance to end up in the spore case.
Lectins’ powers help make sense of how the startling discovery of bacterial farming fits with the revelation of social amoebas’ bacteria-killing immune systems. “Outstanding” work, says Debra Brock of Washington University in St. Louis, who studies both phenomena. “I love mechanisms.”